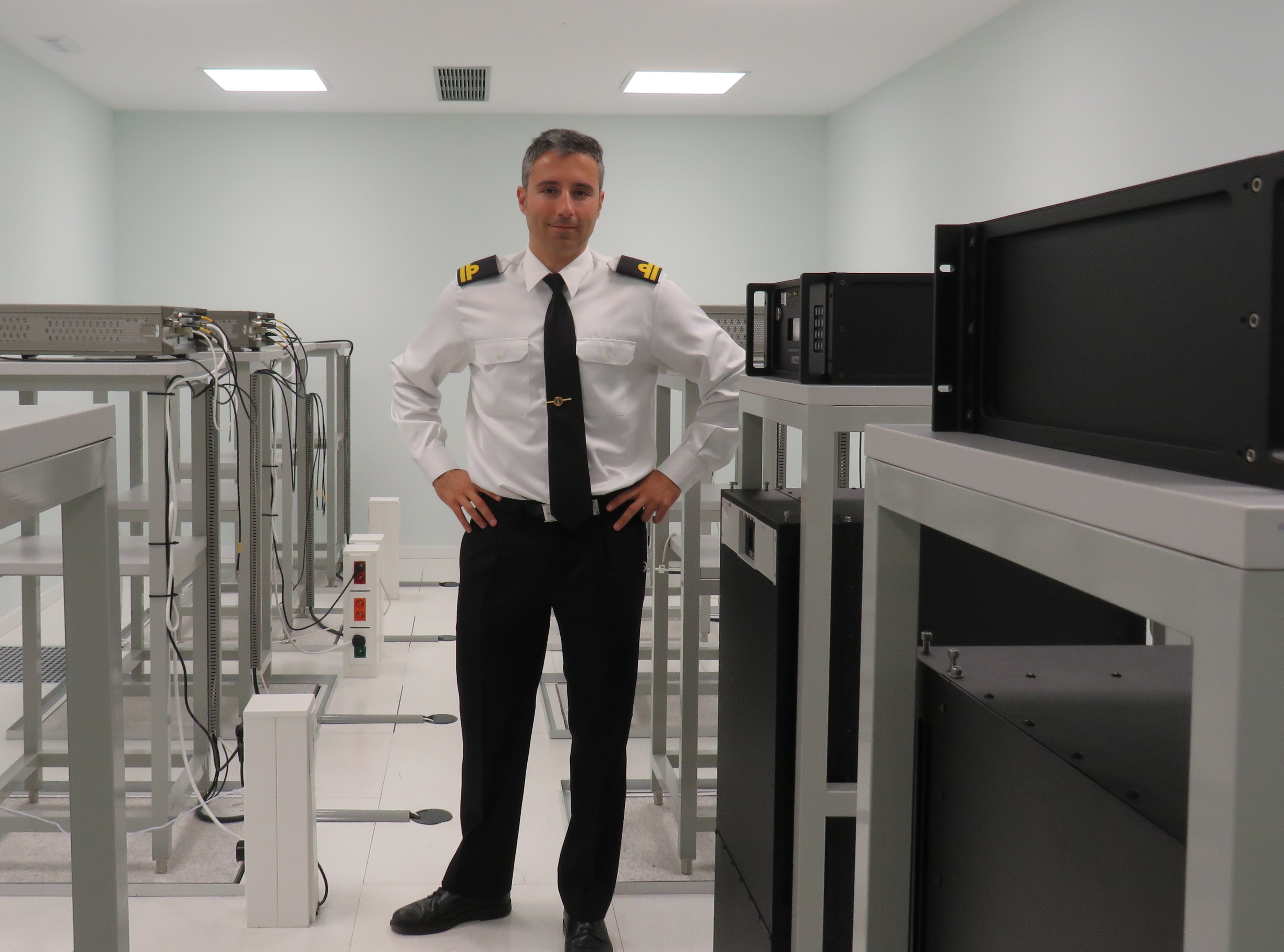
The first phase of the construction of the strontium optical lattice clock by the Royal Institute and Observatory of the Navy – ROA – begins
INTERVIEW HÉCTOR ÁLVAREZ MARTÍNEZ – ROA
At the Royal Institute and Observatory of the Navy in San Fernando, Cadiz, a strontium optical lattice clock is being built, one of the most advanced in the world in this technology. Last November, our company, Ingemation Ingeniería, made the last delivery of the equipment we supplied for the construction of this clock, which is expected to be completed before 2030.
With this delivery, the initial phase of construction begins, known as the optical frequency generation and measurement phase, with the installation of the fundamental equipment to be used, including wavelength meter, optical frequency comb, ultra-stable cavities, vacuum systems, and the start-up of the initial laser systems. In other words, this phase will incorporate lasers that will be used for cooling, confinement, and interrogation of the atoms, which will allow this clock to be of maximum accuracy and stability.
To find out more about this project of great importance for world time, we have conducted an interview with the team leader: Héctor Álvarez Martínez, with a specialisation in physical mathematical sciences and a master’s degree in Astronomy and Geophysics from the Spanish Navy, and a doctorate in physical sciences from the Sorbonne Université (Paris) and the Universidad Autónoma de Madrid.
HOW DO OPTICAL ATOMIC CLOCKS WORK?
This type of clock, as atomic clocks, consists of interrogating several atoms, neutrals, or ions, by means of a main (“imperfect”) oscillator, which will subsequently be corrected thanks to the frequency extracted from the atoms, which will constitute our true (ideal) reference.
The name “optical” is due to the working frequency at which these clocks operate, i.e., both the chosen atomic transition and the oscillator that will interrogate it, which, in this case, will be a laser. Once the atoms are excited by laser interrogation, they will return to their initial energetic state, emitting a fluorescence that will be detected and used as an error signal, which will be our real frequency discriminator.
In this way, we will be able to electronically feedback and correct the laser interrogator, which will ultimately provide a frequency that is now “perfect” for its treatment and subsequent use as a time signal that will mark the ticks of our clock.
YOU HAVE DISTINGUISHED BETWEEN NEUTRAL ATOMS AND IONS, WHAT IS THE DIFFERENCE?
That is, depending on the type of particle used as a reference, there are two different clocks. The basic operating principle, mentioned above, is the same, but the interrogation scheme, its practical implementation and performance are substantially different. On the one hand, we have the ions which, being charged particles, are easier to “trap” by using electric fields with the well-known Paul traps or these together with a magnetic field, which would be the Penning traps.
On the other hand, the strong repulsion experienced by these ions, due to their electrical charge, means that these traps are limited to a single ion (or a few), limiting the stability of the clock. On the other hand, clocks using neutral atoms do not have this limitation, but are more difficult to immobilise for subsequent interrogation.
Capture and cooling are carried out by using a Zeeman slower and one (or two) magneto-optical trap(s) (MOT) as well as the different lasers involved. Finally, the atoms are confined in an optical lattice formed by another laser, like an egg crate, from which the clock gets its name: optical lattice clock. In addition, after each interrogation, new atoms have to be reloaded, captured and confined in the MOT, which further complicates the synchronisation of all the laser systems and electro-optical components involved.
YOUR CIROES PROJECT (CONSTRUCTION AND IMPLEMENTATION OF A STRONTIUM OPTICAL NETWORK CLOCK) IS CURRENTLY UNDERWAY, ALTHOUGH I UNDERSTAND IT IS FOR THE REALISATION OF AN OPTICAL NETWORK CLOCK, WHY IS IT MORE COMPLICATED?
Indeed, its acronym stands for the Construction and Implementation of a Strontium Optical Network Clock. In the ROA, we have opted for such a clock despite its greater complexity and longer implementation time for two reasons. Firstly, these clocks have greater potential in terms of stability. Although it is true that in terms of accuracy both clocks (ion and optical network) are similar, better stability allows the desired accuracy to be achieved in a shorter time, which makes them ideal for metrological time and frequency applications.
It should not be forgotten that the ROA has national responsibility in this area. Secondly, laser atom cooling techniques open the door to a host of new areas of research in quantum physics that could be of interest to the Navy in the years to come.
WHY STRONTIUM?
There is a trade-off between the intrinsic metrological characteristics of the chemical species itself and the technical constraints associated with its handling. For example, we must ensure that laser technology is available or know how well we will be able to characterise and correct for possible deviations in frequency due to both internal and external perturbations to the atoms.
Strontium is a naturally abundant metal that can be evaporated at reasonably high temperatures and belongs to the group of alkaline earth metals, i.e., it has two electrons in its last shell. This gives rise to a series of intercombination lines that provide us with the different optical transitions necessary for both the cooling of the atoms and the excitation of the clock transition.
WHAT HAS CHANGED SINCE THE CREATION OF THE TIME SECTION BY THE NAVY OBSERVATORY IN 1971 UNTIL 2021 WITH THE CONSTRUCTION OF THE STRONTIUM CLOCK?
The Time Section was created with the arrival of the first two atomic clocks, because until then time depended on the Astronomy Section. It was the astronomers who were responsible for determining the time based on the passage of a star (the Sun or a star) through the meridian of the place. The time was based on what is called mean solar time.
Between 1950 and 1954, however, research into microwave spectroscopy began, and in 1955 an atomic clock was demonstrated that was capable of measuring time with greater precision than astronomers were able to determine through observations of the stars. This led to the proliferation of a series of clocks that no longer needed to be corrected by astronomers based on their observations.
Until then, mechanical, pendulum or even quartz clocks were the norm. These clocks, in essence, are the local oscillator of which they are composed: the spiral spring that transmits its energy to the gears, the weights that make the pendulum move, or the vibrations of the quartz itself when subjected to a potential difference. They all try to mark time intervals as uniformly as possible, but they need an external, universal reference so that they can be calibrated and corrected if necessary.
For example, the swinging of the pendulum vertically will vary due to external disturbances, such as temperature and humidity, which will cause small expansions of the material of which the pendulum is made, and which will result in non-periodic oscillations.
It is almost the same with quartz. Mainly because of the temperature, these oscillations (vibrations) start to show fluctuations and are not as constant as we thought. In fact, our wristwatches are designed to work at 35 or 36 degrees Celsius. If we put one of them in a refrigerator, we will see that the next day it may be almost one or two minutes behind or two minutes ahead, depending on the quality of the quartz.
With atomic clocks we forget about the quartz that is corrected by astronomers and now it is corrected by the frequency fluctuations that take place inside matter. Among the different energy levels that we find in an atom, if we are able to interrogate the level of interest, we will obtain an energy (frequency) that is very well defined and that will always be the same. And it is precisely this energy emitted by the atom (after having been previously excited) that we will use for reference in our quartz clock.
HOW DID YOU START THIS PROJECT AND HOW MANY PEOPLE ARE PART OF THE TEAM?
After completing the additional training required by the Navy to work in the scientific sections of the ROA, I was assigned to the Time Section. Shortly afterwards, I was lucky enough to receive a research grant associated with one of the EURAMET (European Association of National Metrology Institutes) projects on optical clocks at the Department of Time-Space Reference Systems (SYRTE) at the Paris Observatory.
What was initially going to be one year became three, and so I was able to do my doctoral thesis at the SYRTE, a world reference in optical frequency metrology. It was during my studies there that we began to give shape to our CIROES project, which had already been in the making for a few years.
There are currently four of us working on the project and two more people are expected to join, although many of us have other responsibilities. The idea is to have at least three full-time members.
HOW IMPORTANT IS TEMPERATURE IN THE FUNCTIONING OF THE STRONTIUM CLOCK?
Our laboratory can maintain a temperature-controlled environment with fluctuations of only +/- 0.5 degrees Celsius. But for the clock we must do much better than that. Therefore, the heart of the clock, i.e., the atom capture and confinement zone in the MOT, is under a high vacuum in which the laser radiation hitting the atoms can slow down and trap the atoms in space. It should not be forgotten that the interrogation transition or “clock transition” is only accessible after the atoms have been successfully immobilised. In other words, our clock will run if and only if the atoms have reached temperatures of the order of micro-Kelvin.
WHY ARE OPTICAL WATCHES CONSIDERED TO BE THE WATCHES OF THE FUTURE?
Today they are already a reality, but they have not yet reached their theoretical limit known as quantum projection noise. This is mainly due to technical limitations such as the realisation of (even more) ultra-stable lasers or more efficient atom loading and interrogation cycles.
Everything seems to be converging and they will have reached their fundamental limit in a few years’ time. However, the current performance of optical clocks exceeds by two orders of magnitude the best microwave atomic clocks (caesium source clocks) on which the basic unit of time, the second, is based, and where the current time and frequency infrastructure resides.
Once these clocks start to be implemented in more and more laboratories, providing a robust and consistent clock network, and we have the necessary optical means of comparison on a global scale, these clocks will replace the current microwave clocks without any doubt.
HOW DO THE ROA ATOMIC CLOCKS COMPARE?
The current atomic clocks generate the time scale that we broadcast on the national territory and work in the microwave voucher. Then there is the new generation of clocks we are building, which are still atomic, but are now optical, i.e., they work at faster and higher frequencies. Visible optical frequencies provided initially by a laser. They will be highly accurate and stable clocks.
WHAT REFERENCE SCALE IS USED?
In the ROA, we generate the local Coordinated Universal Time scale, UTC (ROA), which is generated thanks to the microwave frequency standard atomic clocks that are in operation right now since 1972, when the first ones arrived.
This scale is generated by an algorithm that combines information from a battery of atomic frequency standards, consisting of two active hydrogen masers and five caesium beam standards, optimising stability, and providing other metrological features of interest, such as reliability or accuracy control.
The ROA participates with these seven standards in the generation of the International Atomic Time (TAI) scale. We compare our time data with those issued by other international metrological centres, and in turn, other centres use our data to compare the reliability and performance of their oscillators.
WHAT DO YOU MEAN WHEN YOU SAY THAT OPTICAL CLOCKS “WILL NOT MOVE FORWARD OR BACKWARD BY ONE SECOND FOR 15 BILLION YEARS”?
It is a rough calculation that accounts for the relative uncertainty of an optical clock, which can be as much as 1 part in 1018, in a more intuitive and easy-to-remember way. If we consider the approximately 5×1017 seconds contained in those 15 billion years, which is a figure close to the age of the universe, this would be equivalent to saying that our clock would make an error of less than one second if it had been started since then.
ARE OPTICAL CLOCKS ALSO USEFUL IN GEODESY AND DO THEY ALLOW MORE DETAILED MEASUREMENTS OF THE SHAPE OF THE EARTH?
Absolutely. Instead of using the knowledge we have about the earth’s gravitational field to predict the changes in frequency between two distant clocks, we can turn the problem around and see if the change in frequency of these can improve our knowledge of the gravitational field.
Roughly speaking, according to general relativity, one centimetre equals a change in relative frequency of 10-18, so if we can compare clocks with these accuracies, we can carry out studies of altimetry and gravimetry to levels never seen before, a discipline recently termed “chronometric geodesy”.
IS IT TRUE THAT THE STRONTIUM WATCH IS SO SENSITIVE THAT IT CAN DETECT ELEVATION CHANGES AS SMALL AS PLACING A SMALL BOOK UNDER THE WATCH?
To date, frequency comparisons of optical clocks with uncertainties of the order of 10-18 have been demonstrated, including strontium, so it would be detectable. As you say, this means that we can perfectly discern changes in height between the two clocks under comparison of the order of a few centimetres.
INGEMATION ENGINEERING HAS COMPLETED THE LAST DELIVERY OF THE 2021 EXERCISE. WHAT STAGE OF CONSTRUCTION ARE YOU CURRENTLY AT?
The CIROES project started at the end of 2019 and has been conceived in a total of four phases, one per year, in terms of procurement of material, equipment and systems for the implementation of the clock. We have recently received the material corresponding to the third and penultimate delivery.
Naturally, these acquisitions have been programmed to follow the logical order of implementation, but they do not necessarily have to go hand in hand. In fact, the achievement of the technical and scientific objectives will take considerably longer.
With this last delivery of the year, we start the initial phase of the clock construction, with the commissioning of the main equipment to be used, such as the wavelength meter, the optical frequency comb, ultra-stable cavities, and the initial laser systems. Let’s call it the optical frequency generation and measurement phase.
IN THIS LATEST RELEASE, AN ULTRA-STABLE CAVITY WITH CRYSTALLINE MIRRORS WILL BE INCORPORATED, WHAT IS ITS FUNCTION?
Among all the lasers that will be part of the optical clock, where more than seven different optical frequencies will be involved, there is one that requires special attention: the clock laser. This red laser (at 698 nm) will oversee probing and interrogating the atoms once they are confined in the optical lattice. To do this, this laser must be ultra-stable, i.e., its frequency must be as monochromatic as possible and fluctuate as little as possible over time in the short term (say from one second to the next). This is achieved by stabilising the initial laser in a Fabry-Perot type ultra-stable optical linear cavity.
In essence, the cavity is made up of two extremely reflective mirrors (known as crystalline) mounted on a low thermal expansion crystal that is enclosed in a high vacuum system. This in turn rests on an anti-vibration platform and is suitably insulated both thermally and acoustically.
In this way, the initial laser light injected into the cavity (the mirrors allow a small part to be transmitted through them) is filtered and coupled in one of its resonant modes. Using electronic techniques, an error signal is generated and used to feedback and correct the laser, eliminating its fluctuations at source and making it more stable.
WHEN IS THE CONSTRUCTION OF THE CLOCK SCHEDULED TO BE COMPLETED?
I would not know how to answer this question with certainty. Initially we set ourselves the goal of achieving it in 6 years (starting in 2020) but we are a year behind schedule, partly due to the pandemic. It depends on many variables, for example that we do not suffer delays in the purchase of very specific optical material due to a lack of raw materials, as is happening to us right now, or that a technical problem arises in some equipment and it has to be sent back to the manufacturer to replace a part, as has also happened to us recently.
We are talking about a non-commercial laboratory clock, which is complex to build and operate, and which has been implemented to date only in the world’s leading scientific time and frequency metrology laboratories.
Our goal is to have it ready by 2030
WHAT WILL THE WATCH LOOK LIKE?
It will consist of three optical tables. In the first one we will have the laser systems that generate the frequency. The second one will contain the heart of the clock where the magneto-optical trap that will capture and cool the atoms, and the optical network where they will be confined and interrogated.
And finally, there would be a third table for the measurement and characterisation of all the lasers involved. In total, we will work with seven different frequencies and around twenty perfectly matched lasers.
CAN WE SAY THAT YOU ARE THE MASTERS OF STATE-OF-THE-ART WATCHES?
Yes, something like that. Master watchmakers of the 21st century.